Bioeconomy discussions are typically centred around carbon. How to reduce carbon emissions? How to increase soil carbon? What about carbon sequestration? How do we support low carbon fuel development? These are all common questions.
In recent years there’s been a newcomer to these discussions, hydrogen has entered the debate with proponents and detractors questioning the potential and value of a future hydrogen economy.
However, the most abundant element on earth and the second most abundant in biomass, is rarely a topic of conversation. The reason behind this lies in the focus of the bioeconomy on the supply of biofuels and bioenergy.
Fuel and energy
The production of liquid biofuels has a focus on replicating and displacing fossil fuels used in transport e.g. petrol and diesel in road transport, bunker fuel for shipping and jet fuel in aviation.
All fossil fuels are hydrocarbons, composed of carbon and hydrogen, simply varying in the carbon to hydrogen ratio or the way in which the carbons are connected. What all fossil fuels have in common is an absence of oxygen.
The presence of oxygen in biofuels such as ethanol and fatty acid methyl esters (FAME) is regarded as a detriment to the fuel, reducing calorific content and the cause of issues with fuel stability or hygroscopicity (i.e. their tendency to absorb water). However, oxygen does play an important role in fuel additives such as ethanol, where its presence enhances fuel combustion and thereby reduces exhaust emissions. The presence of oxygen in some biofuels creates challenges in blending these fuels with fossil fuels, creating what is known as a blend wall. To overcome these issues, biofuels development has focussed on the production of fungible fuels where the oxygen in biomass feedstock is removed. As a result the biofuel can now theoretically be blended up to 100% with fossil fuel. For example the production of hydrogenated vegetable oil (HVO) is favoured over the production of FAME as a diesel replacement.
So, is oxygen the villain hidden in biomass feedstock? Maybe from an energy perspective but quite a different story develops when considering the production of biobased chemicals and materials.
Chemicals and materials
Starch and cellulose are key biomass intermediates in the production of both biofuels and materials. However, whereas fuel production looks to reduce or remove the oxygen in starch and cellulose, materials such paper, starch-based adhesives and plastics have no issues with its presence.
In the petrochemical industry, which provides the inputs for 96% of all the products we use, considerable
effort and energy is expended towards adding oxygen to the hydrocarbon based raw materials (coal,
oil and fossil gas) that it uses. The presence of oxygen in biomass feedstock creates a potential
opportunity for biobased chemical production to by-pass fossil-derived primary chemicals to directly
produce oxygenated chemical intermediates (Figure 1).
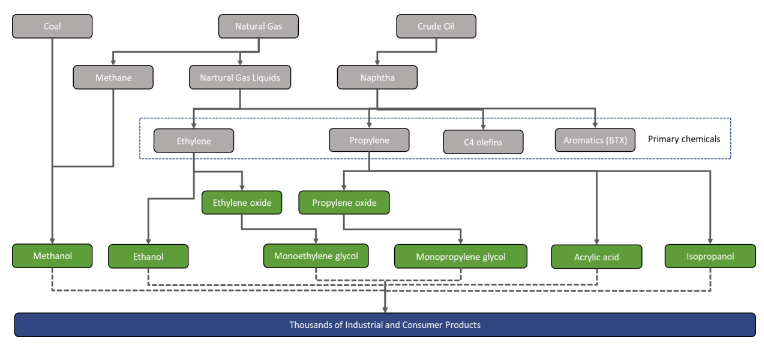
(green boxes)
Economics and Land
How we view and value oxygen also impacts on process economics and the land required to make chemicals and polymers.
It is possible to recreate the petrochemical value chain based on biomass feedstock, i.e. converting biomass to the key primary chemicals, ethylene, propylene etc. (Figure 1.). However, in doing this we risk suffering from a poor mass efficiency/balance as we eliminate oxygen (as water or carbon dioxide). This consideration can be demonstrated by comparing the production of the three polymers, cellulose polylactic acid and polyethylene.
Based on a reasonable biomass yield of 10 tonnes per ha, a theoretical yield of four tonnes of cellulose polymer could be produced. This assumes no process losses in the conversion of biomass to cellulose which is of course unrealistic. The same yield holds true for polylactic acid, the four tonnes of cellulose can be converted to lactic acid (2 moles of lactic acid produced per mole of glucose monomer, equation
1) and polymerised to polylactic acid with a theoretical hundred percent mass yield.
𝐸𝑞 1𝑎. 𝐶𝑒𝑙𝑙𝑢𝑙𝑜𝑠𝑒 𝑡𝑜 𝑔𝑙𝑢𝑐𝑜𝑠𝑒 𝑡𝑜 𝑙𝑎𝑐𝑡𝑖𝑐 𝑎𝑐𝑖𝑑 𝑡𝑜 𝑝𝑜𝑙𝑦𝑙𝑎𝑐𝑡𝑖𝑐 𝑎𝑐𝑖𝑑
𝐶6𝐻10𝑂5 + 𝐻2𝑂 → 𝐶6𝐻12𝑂6 → 2 𝑥 𝐶3𝐻6𝑂3 → 𝐶6𝐻10𝑂5 + 𝐻20
𝐸𝑥𝑝𝑟𝑒𝑠𝑒𝑑 𝑎𝑠 𝑓𝑜𝑟𝑚𝑢𝑙𝑎 𝑤𝑒𝑖𝑔ℎ𝑡𝑠: 162𝑔 + 18𝑔 → 180𝑔 → 2 𝑥 90𝑔 → 162𝑔 + 18𝑔
However producing ethylene and polyethylene requires deoxygenation. This means that assuming, all the carbon contained in cellulose could be converted to ethylene (i.e. 3 moles of ethylene per mole of glucose, equation 2) we would lose 48% of starting mass through the loss of oxygen or in other words our four tonnes of cellulose would theoretically yield a little over 2 tonnes of polyethylene. Critically, twice as much biomass is required to produce an equivalent amount of polyethylene to polylactic acid and therefore twice as much land is theoretically required.
𝐸𝑞 2. 𝐶𝑒𝑙𝑙𝑢𝑙𝑜𝑠𝑒 𝑡𝑜 𝑔𝑙𝑢𝑐𝑜𝑠𝑒 𝑡𝑜 𝑒𝑡ℎ𝑦𝑙𝑒𝑛𝑒 𝑡𝑜 𝑝𝑜𝑙𝑦𝑒𝑡ℎ𝑦𝑙𝑒𝑛𝑒
𝐶6𝐻10𝑂5 + 𝐻2𝑂 → 𝐶6𝐻12𝑂6 → 3 𝑥 𝐶2𝐻4 → 𝐶2𝐻4
𝐸𝑥𝑝𝑟𝑒𝑠𝑠𝑒𝑑 𝑎𝑠 𝑓𝑜𝑟𝑚𝑢𝑙𝑎 𝑤𝑒𝑖𝑔ℎ𝑡𝑠: 162𝑔 + 18𝑔 → 180𝑔 → 3 𝑥 28𝑔 → 84𝑔
The difference in mass efficiencies also impacts on production economics given the need to procure significantly larger quantities of biomass to produce polyethylene compared to polylactic acid.
Of course, differing process yields complicates the situation, but this difference demonstrates the need to consider mass efficiency and land requirements when considering the potential for chemical and polymer production and their environmental impacts.
Life Cycle Analysis
Life cycle analysis (LCA) is widely used to assess the environmental impacts of producing a product. Such analysis can apply various methods of allocating environmental burdens between process intermediates, co-products and by-products. Two common methods of allocation are by energy or mass content. The impacts of this on the environmental burden borne by each co-product can be significantly affected by the approach adopted. Differences seen in LCA results can directly relate to views taken on the value of oxygen in either providing mass, or its lack of value in providing energy. There may be no conflict in this view, for example if only considering fuel production. However, if both fuel and chemical products (consider the coproduction of ethanol and carbon dioxide) are being produced then careful thought and explanation of choice of allocation method will be required to avoid unintentionally burdening one or other output detrimentally compared to comparator materials. Alternatively other methods of apportioning environmental burdens, such as price allocation or by using product credits, may be more appropriate.
Final remark
So, the next time you’re discussing biomass and its potential uses, give a thought to oxygen and whether we’re making the best use of all the elements in the feedstock.
Author
Adrian Higson
Source
NNFCC, press release, 2024-02-01.
Supplier
Share
Renewable Carbon News – Daily Newsletter
Subscribe to our daily email newsletter – the world's leading newsletter on renewable materials and chemicals